The 20 TW peak power CPA hybrid Ti:sapphire/Nd:phosphate glass system delivers 8 J in 400 fs with focused intensity up to 3.1019 W/cm2 (λ at 1.053 μm) with the ASE energy contrast of better than 10-5. After the pulse compression the laser is sent to the plasma mirror chamber, where contrast is improved by another 2.5 orders of magnitude. After that the pulse is delivered to the experimental chamber, equipped with vacuum pumps, target positioning system and optics. In the past this laser has been successfully used for the discoveries of many high-field science effects, such as wakefield electron acceleration and observation of collimated electron beam [1], observation of nonlinear Thomson scattering [2], observation of Coulomb explosion and ion acceleration in underdense plasma [3], observation of proton acceleration from thin film targets [4], radioactive isotope production with accelerated ions [5], observation of relativistic harmonics generation [6] and others. More recently the T-cubed laser was used to study the front vs rear light ion acceleration [7], for development of a high purity deuteron beams [8], neutron source generation [9], study of the proton conversion efficiency in isolated reduced mass targets [10], and investigation of hot electron dynamics via copper Kα imaging [11]
The T-cubed oscillator system “Mira-900” by Coherent utilizes a Kerr mode-locked Ti:sapphire laser producing a 76 MHz train of 100 fs pulses operating at a wavelength of 1.053 microns with an average power of 200 mW. This oscillator is pumped with CW DPSS Sprout-G green laser from Lighthouse Photonics with an average power of ~10 W. The short pulse train from the oscillator is sent to the stretcher, where it is stretched out temporally by 4 passes on a single 1740 lines/mm grating to approximately 1 ns (Fig. 1). After the selection of a single pulse with the Pockels cell at 10 Hz repetition rate it is amplified in the Ti:sapphire regenerative amplifier to a 1 mJ level. The regenerative amplifier is pumped with frequency doubled 10 Hz repetition rate Quantel “Smart” laser. The pump laser has wavelength of 0.53 micron, duration of 6 ns and delivers ~30 mJ/arm of pump energy to both ends of Ti:sapphire crystal.
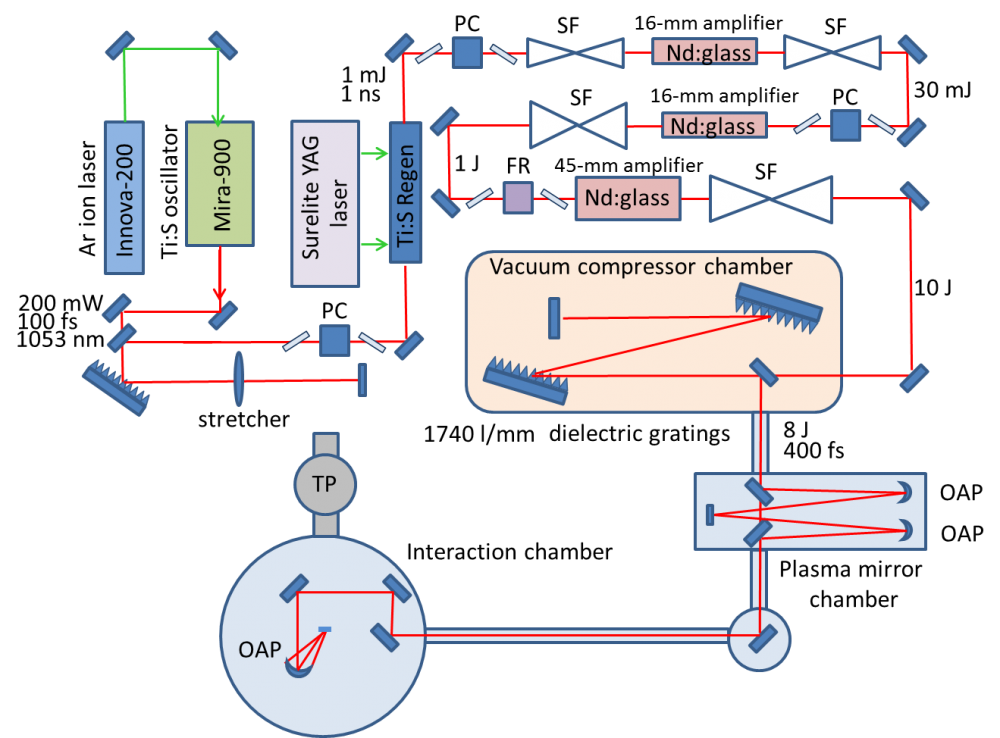
Fig.1 Schematic of T-cubed 15 TW, 400 fs laser
The regen output is coupled into the amplification chain, where it is amplified in Neodymium doped glass rod (Nd:Glass) amplifiers up to 10 Joules. The chain currently consists of two heads with rods of 16×500 mm and one head with a rod of 45×500 mm. Vacuum spatial filters after each Nd:Glass amplifier vacuum are used for beam expansion, relay imaging and beam smoothing by elimination of local modulations of its intensity. The operational repetition rates of the final amplifiers are 3 min for the 16 mm heads and 8 min for the 45 mm head. The amplified pulse is delivered to the vacuum compressor chamber (Fig. 2), where it is compressed by a grating pair (1740 l/mm) to duration of 400 fs and has a maximum energy of 8 J. The 10 Hz Ti:Sapphire regenerative amplifier is used as an alignment laser for the amplifier chain, gratings pulse compressor and the experimental setup. From the output of the compressor chamber the beam is delivered to the plasma mirror chamber, where the ASE intensity contrast is improved to better than 10-7 and later to the experimental chamber. The pulse is focused on a solid or gas target to produce high-temperature relativistic plasmas.
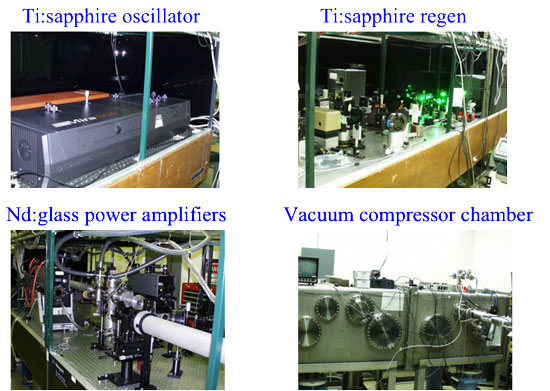
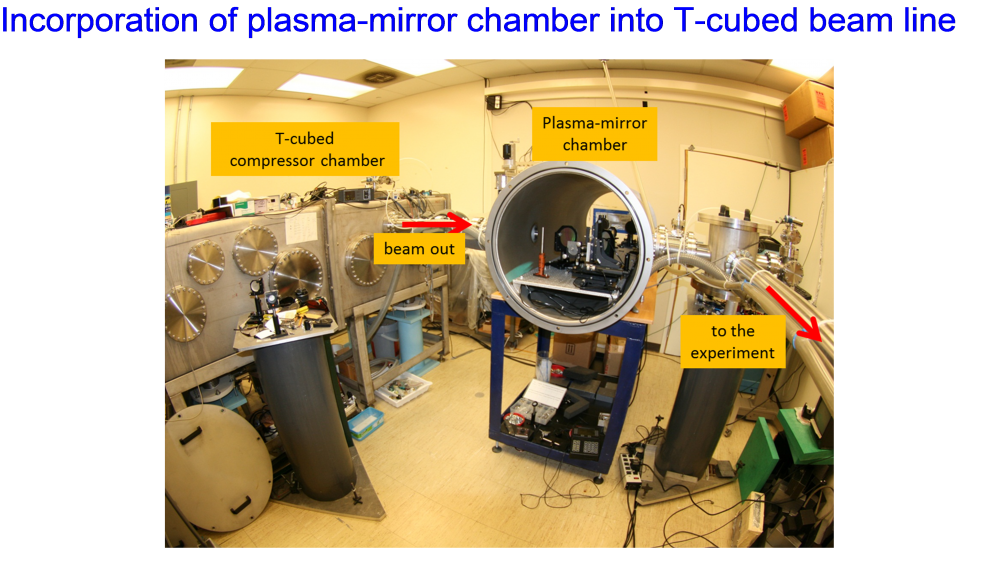
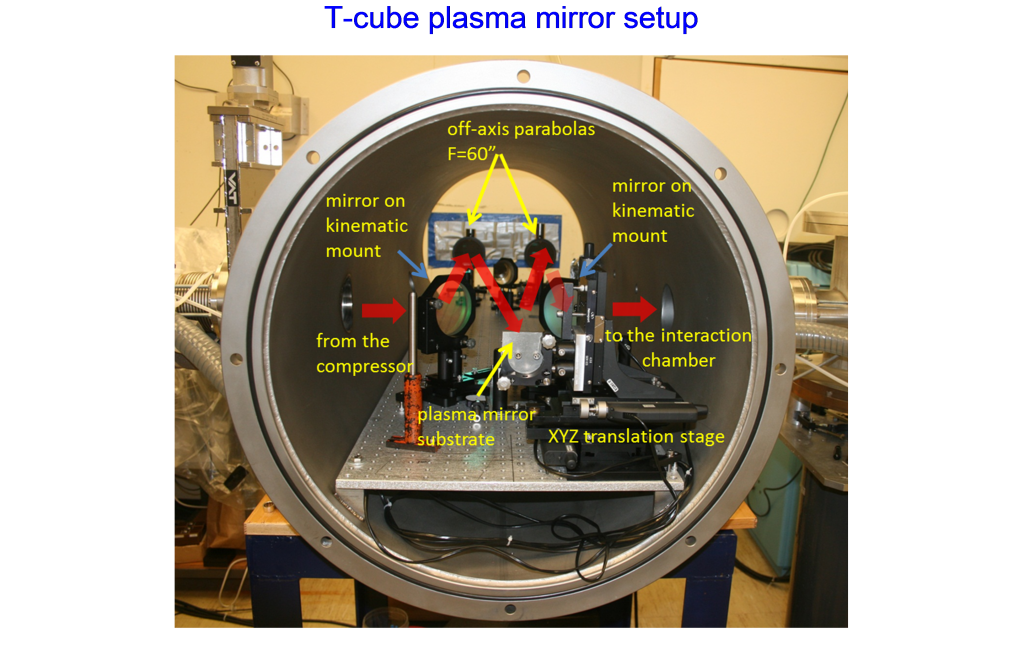
Fig.2 Elements of T-cubed laser
References:
- D. Umstadter, S.-Y. Chen, A. Maksimchuk, G. Mourou, and R. Wagner, “Nonlinear optics in relativistic plasmas and laser wakefield acceleration of electrons,” Science 273, 472 (1996).
- S.-Y. Chen, A. Maksimchuk and D. Umstadter, “Experimental observation of relativistic nonlinear Thomson scattering,” Nature 396, 653 (1998).
- G. S. Sarkisov, V. Yu. Bychenkov, V. N. Novikov, V. T. Tikhonchuk, A. Makismchuk, S. -Y. Chen, R. Wagner, G. Mourou and D. Umstadter, “Self-focusing, channel formation and high-energy ion generation in interaction of an intense short laser pulse with a He jet,” Phys. Rev. E 59, 7042 (1999).
- A. Maksimchuk, S. Gu, K. Flippo, D. Umstadter and V. Yu. Bychenkov, “Forward ion acceleration in thin films driven by a high-intensity laser,” Phys. Rev. Lett. 84, 4108 (2000).
- K. Nemoto, A. Maksimchuk, S. Banerjee, K. Flippo, G. Mourou, D. Umstadter and V. Bychenkov, “Laser-triggered ion acceleration and table top isotope production,” Appl. Phys. Lett. 78, 595 (2001).
- S. Banerjee, A. R. Valenzuela, R. C. Shah, A. Maksimchuk, and D. Umstadter, “High harmonic generation in relativistic laser plasma interaction,” Phys. Plasmas 9, 2393 (2002).
- L. Willingale, G. M. Petrov, A. Maksimchuk, J. Davis , R. R. Freeman, T. Matsuoka, C. D. Murphy, V. M. Ovchinnikov, L. Van Woerkom and K. Krushelnick, “Front versus rear side light-ion acceleration from high-intensity laser–solid interactions,” Plasma Phys. Control. Fusion 53, 014011 (2011).
- A. Maksimchuk, A. Raymond, F. Yu, G. M. Petrov, F. Dollar, L. Willingale, C. Zulick, J. Davis, and K. Krushelnick, “Dominant deuteron acceleration with a high-intensity laser for isotope production and neutron generation,” Appl. Phys. Lett. 102, 191117 (2013).
- L. Willingale, G. M. Petrov, A. Maksimchuk, J. Davis, R. R. Freeman, A. S. Joglekar, T. Matsuoka, C. D. Murphy, V. M. Ovchinnikov, A. G. R. Thomas, L. Van Woerkom, and K. Krushelnick, “Comparison of bulk and pitcher-catcher targets for laser-driven neutron production,” Phys. Plasmas 18, 083106 (2011).
- A. Morace, C. Bellei, T. Bartal, L. Willingale, J. Kim, A. Maksimchuk, K. Krushelnick, M. S. Wei, P. K. Patel, D. Batani, N. Piovella, R. B. Stephens, and F. N. Beg, “Improved laser-to-proton conversion efficiency in isolated reduced mass targets,” Appl. Phys. Lett. 103, 054102 (2013).
- L. Willingale, A. G. R. Thomas, A. Maksimchuk, A. Morace, T. Bartal, J. Kim, R. B. Stephens, M. S. Wei, F. N. Beg, and K. Krushelnick, “Investigation of relativistic intensity laser generated hot electron dynamics via copper Kα imaging and proton acceleration,” Phys. Plasmas 20, 123112 (2013).