HERCULES 300 TW laser
The construction and operation of a high-field petawatt class laser, HERCULES, is a major CUOS activity. The National Science Foundation through the Physics Frontier Center FOCUS supported the development and construction of this laser. The goal of High-Field Science program at CUOS is to explore the ultra-relativistic intensity regime of laser-matter interaction. The Petawatt stage of HERCULES was activated in 2007 and reached power of 300 TW [1]. This was the first multi-100 TW-scale repetitive laser. HERCULES holds world records for the highest focused intensity, 2×1022 Wcm-2 and for Amplified Spontaneous Emission (ASE) temporal contrast of 10-11.
The HERCULES laser design is based on chirped-pulse amplification with cleaning of amplified spontaneous emission (ASE) noise after the first amplifier (Fig. 1).The output pulse of the short pulse oscillator (12 fs-pulsewidth, Femtolasers) of the HERCULES laser is preamlified in the two-pass preamlifier to the microjoule energy level. ASE added by the two-pass amplifier is removed by the cleaner based on cross-polarized-wave generation [2] providing a record ASE contrast of 10-11 [3]. The clean microjoule energy pulse is stretched to ~0.5 ns by the stretcher based on a modified mirror-in-grating design [4]. The whole laser is designed by ray-tracing analysis to be fifth-order dispersion-limited over 104 nm bandwidth. The high-energy regenerative amplifier [5] and cryogenically cooled 4-pass amplifier bring the pulse energy to a joule energy level with nearly diffraction-limited beam quality. Two sequential 2-pass-Ti:sapphire amplifiers of 1′ and 2″ beam diameter respectively raise the output energy to a value approaching 20 J.
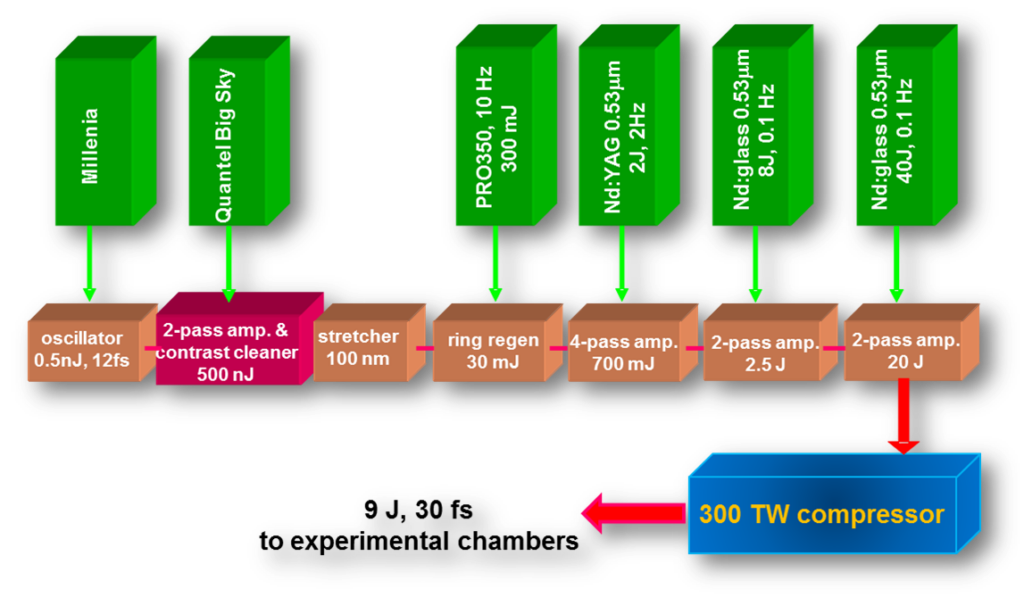
We designed our own frequency-doubled Nd:glass pump laser [6] for pumping of the final two amplifiers of the HERCULES laser (Fig. 2). The pump laser has two stages of amplification. The frequency-doubled output of the first stage is used for pumping of the 1″-diameter Ti:sapphire amplifier, while the unconverted infrared light is injected into the second stage of the pump laser for further amplification. The frequency-doubled output of the second stage is used for pumping of the booster (2″- diameter) amplifier of the HERCULES laser. The pump laser has a quasi-flat-top beam profile that was achieved at 0.1 Hz repetition rate by relay imaging and thermally-introduced birefringence compensation. The booster two-pass amplifier uses a 11-cm-diameter Ti:sapphire crystal. Only a portion of this crystal is used to amplify the 2″ – diameter output beam of the HERCULES laser. In order to suppress parasitic oscillations the side surface of the crystal is covered with a thin layer of index-matching thermoplastic coating (Cargille Laboratories, Inc.) doped with organic dye absorbing at 800 nm.
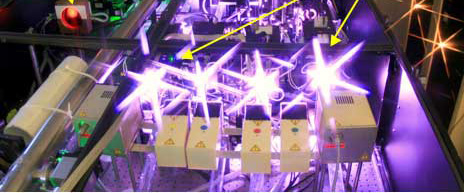
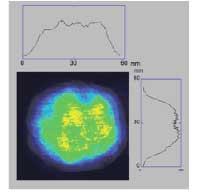
The output beam profile (Fig. 3) is quasi
-flat-top as a result of using flat-top pump beams and of the image relaying of the amplified beam through the whole laser chain. Output energy of 17 J corresponding to 300 TW power after compression has been reached so far. The pump energy for the booster Ti:sapphire amplifier (2″-diameter) is controlled by changing the pumping level of the oscillator of the pump laser.
The output pulse is compressed in a 4-grating compressor [7] to ~30 fs (Fig. 4). The compressor is based on two 42×21 cm-size and two 22×16.5 cm-size 1200 l/mm-gold-coated holographic gratings (Jobin Yvon).
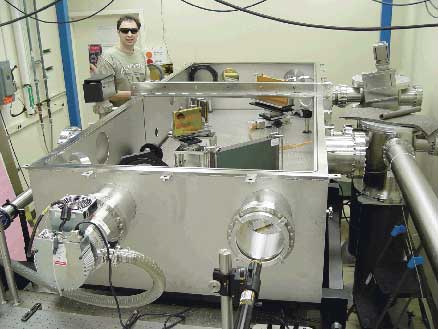
Fig.4 Hercules Petawatt Compressor
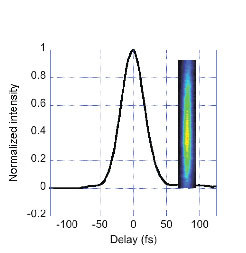
Fig. 5. Autocorrelation of 300 TW pulse showing duration of 30 fs (FWHM). The experimental autocorrelation picture (insert) demonstrates that there is no amplitude front tilt or other spatial variations of the pulse arrival time.
Because the beam size in the compressor is rather large (6″-diameter) achromatic lenses are used in the final relays to prevent spatially varying group delay across the beam. The pulse width is measured at full energy using beam leak-through a mirror by two methods: autocorrelator with inversion [8] (Fig. 5) – to ensure that there is no spatially varying pulse delay, and a single-shot spectral interferometry for direct electric field reconstruction (SPIDER) which was not sensitive to spatial variation of delay but was able to provide phase information for intensity reconstruction. After the beam compression it is down-collimated by the all-reflective telescope to 4″-diameter and is sent to the interaction chamber where it is focused by a parabolic mirror.
Before the parabolic mirror we use a deformable mirror (4″-diameter, 177 actuators, dielectric coated at 800 nm, made by Xinetics) to compensate the aberrations of the parabolic mirror, astigmatism of the telescope and the residual aberrations of the laser beam. The focal distribution is characterized by using the method that we developed in [9,10]. We corrected the wavefront after the f/1 parabola and reached phase aberration (r.m.s.) of lambda/20 (Fig. 6a) leading to the nearly diffraction limited spot (Fig. 6b,c).
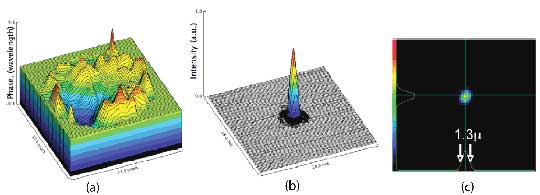
Fig. 6: Focal spot characterization: a) Low-energy-beam wavefront corrected by the deformable mirror, phase aberrations r.m.s. =0.034*lambda, P.V.=0.24l*lambda; b) Intensity distribution in the focal spot of parabolic mirror calculated for the corrected wavefront shown in (a); c) Measured focal spot for a reference low-energy beam focused by f/1 parabolic mirror for the corrected wavefront showing spot size of 1.3 micron (FWHM).
By upgrading HERCULES’s laser power to 300 TW we demonstrated the highest focused intensity to date of ~2×1022 W/cm2. This intensity can be raised to 5×1022 W/cm2 by using a f/0.6 parabolic mirror (as we did in [9]) opening the radiation-dominated regime of electron-light interaction for experimental studies.
References:
1. V. Yanovsky, V. Chvykov, G. Kalinchenko, P. Rousseau, T. Planchon, T. Matsuoka, A. Maksimchuk, J. Nees, G. Cheriaux, G. Mourou and K. Krushelnick, “Ultra-high intensity 300 TW laser at 0.1 Hz repetition rate,” Optics Express 16, 2109 (2008).
2. A. Jullien, O. Albert, F. Burgy, G.Hamoniaux, J.P. Rousseau, J.-P. Chambaret, F. AugERochereau, G. Chériaux, J. Etchepare, N. Minkovski, S.M. Saltiel,”10-10 temporal contrast for femtosecond ultraintense lasers by cross-polarized wave generation,” Opt. Lett. 30, 920-922 (2005).
3. V. Chvykov, P. Rousseau, S. Reed, G. Kalinchenko, and V. Yanovsky, “Generation of 1011 contrast 50 TW laser pulses,” Opt. Lett. 31, 1456-1458 (2006).
4. P. S. Bank, M.D. Perry, V. Yanovsky, S. N. Fochs, B.C. Stuart, and J. Zweiback “Novel All-Reflective Stretcher for Chirped-Pulse Amplification of Ultrashort Pulses” IEEE J. Quant. Electr. 36, 268-274 (2000).
5. V. Yanovsky, C. Felix , and G. Mourou, “High-energy Broadband Regenerative Amplifier for Chirped-pulse Amplification” IEEE J. Sel. Top. Quant. Electr.” 7, 539-541 (2001).
6. V.Yanovsky, V. Chvykov, S.-W.Bahk, G. Kalintchenko, K. TaPhuoc, Y-C. Chang and G.Mourou, “Development of Petawatt scale Ti:sapphire laser at 0.05 Hz repetition rate”, CLEO’2003, paper CME6
7. M. Aoyama, K. Yamakawa, Y. Akahane, J. Ma, N. Inoue, H. Ueda, and H. Kiriyama, “0.85-PW, 33-fs Ti:sapphire laser,” Opt. Lett. 28, 1594-1596 (2003).
8. Z Sacks, G. Mourou, R. Danielius, “Adjusting pulse-front tilt and pulse duration by use of a single-shot autocorrelator,” Opt. Lett. 26, 462-464, (2003).
9. S.-W. Bahk, P. Rousseau, T. Planchon, V. Chvykov, G. Kalintchenko, A. Maksimchuk, G. Mourou, V. Yanovsky, “The generation and characterization of the highest laser intensity (1022W/cm2),” Opt. Lett. 29, 2837-2839 (2004).
10. S.-W. Bahk, P. Rousseau, T. A. Planchon, V. Chvykov, G. Kalintchenko, A. Maksimchuk, G. A. Mourou, V. Yanovsky,” Characterization of focal field formed by a large numerical aperture paraboloidal mirror and generation of ultra high intensity (1022 W/cm2),” Appl. Phys. B 80, 823-832 (2005).